4,6-Dimethylpyrimidine-2-thiol hydrochloride (1) was synthesized by a reaction of thiourea with acetylacetone catalyzed by conc. HCl [6]. Benzoylation of compound 1 with derivatives of benzoyl chloride [6] gave pyrimidine benzothioate derivatives (2–5). Sulfoxidation of compounds 2–5 by hydrogen peroxide and glacial acetic acid gave derivatives of pyrimidine sulfonyl methanone (6–9) (Fig. 1).
Sulfoxidation of pyrimidine benzothioate derivatives.
Characterization of pyrimidine sulfonyl methanone derivatives (6–9)
Sulfoxidation of (4,6-dimethylpyrimidin-2-yl)benzothioate (2), (4,6-dimethylpyrimidin-2-yl)-4-methyl benzothioate (3), (4,6-dimethylpyrimidin-2-yl)-4-chlorobenzothioate (4) and (4,6-dimethyl pyrimidin-2-yl)-4-nitrobenzothioate (5) by hydrogen peroxide and glacial acetic acid afforded (4,6-dimethylpyrimidin-2-yl)sulfonyl)(phenyl)methanone (6), 4,6-dimethyl pyrimidin-2-yl)sulfonyl) (p-tolyl)methanone (7), (4-chlorophenyl)((4,6-dimethylpyrimidin-2-yl)sulfonyl)methanone (8) and ((4,6-dimethylpyrimidin-2-yl)sulfonyl)(4-nitrophenyl) methanone (9), respectively.
FT-IR spectra of compounds (6–9) showed the stretching carbonyl groups at 1690, 1679, 1685, and 1696 cm−1 and the sulfonyl groups at 1324, 1283, 1317 and 1292 cm−1, respectively (Fig. 2). 1H-NMR spectrum (CDCl3) of compound 6 illustrated the chemical shift (δ = ppm) at 2.48 (s, 6H, pyrimidine methyl protons), at 7.43 (s, 1H, aromatic protons of pyrimidine ring) and 7.45–7.91 (m, 5H, aromatic protons). 13C NMR (CDCl3) δ(ppm) of compound 6: 188.22, 171.11, 163.41, 149.63, 139.82, 134.35, 131.58, 124.24 and 29.33. The mass spectrum of compound 6 (Fig. 3) showed the molecular ion peak at 276.02 (M+, 3.02%). Additionally, 1H-NMR spectrum (CDCl3) of compound 7 the chemical shift (δ = ppm) at 1.2 (s, 3H, tolyl methyl protons), at 2.48 (s, 6H, pyrimidine methyl protons), at 7.24 (s, 1H, aromatic protons of pyrimidine ring) and 7.27–7.82 (m, 4H, aromatic protons). 13C NMR (CDCl3) δ(ppm) of compound 7: 188.88, 165.11, 158.47, 139.64, 133.82, 129.35, 126.58, 119.24, 33.39 and 19.32. Moreover, the 1H-NMR spectrum (CDCl3) of compound 8 illustrated (s, 6H, pyrimidine methyl protons) at 2.47, (s, 1H, aromatic protons of pyrimidine ring) at 7.51 and (m, 4H, aromatic protons) at 7.53–7.91 ppm. 13C NMR (CDCl3) δ(ppm) of compound 8: 192.12, 174.14, 167.51, 148.65, 141.82, 137.97, 131.78, 123.99 and 29.39. 1H-NMR spectrum (CDCl3) of compound 9 illustrated (s, 6H, pyrimidine methyl protons) at 2.47, (s, 1H, aromatic protons of pyrimidine ring) at 7.41 and (m, 4H, aromatic protons) at 7.65–8.35 ppm. 13C NMR (CDCl3) δ(ppm) of compound 9: 191.52, 173.25, 166.55, 147.66, 141.82, 137.75, 119.22, 114.19 and 24.34. The mass spectrum of compound 9 (Fig. 4) showed the molecular ion peak at 321.41 (M+, 0.07).
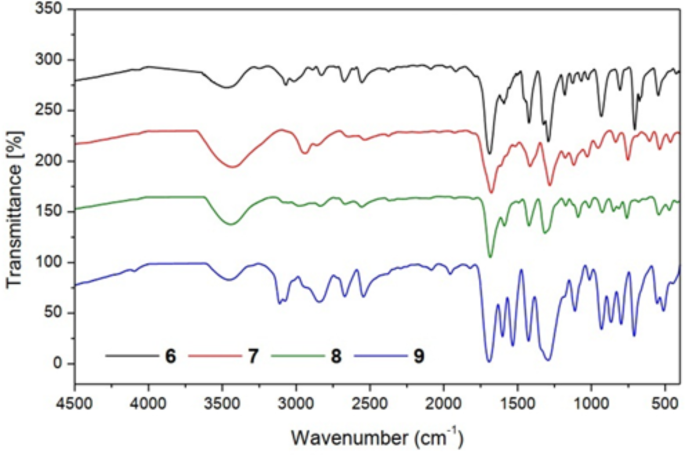
FT-IR spectra of pyrimidine sulfonyl methanone derivatives (6–9).
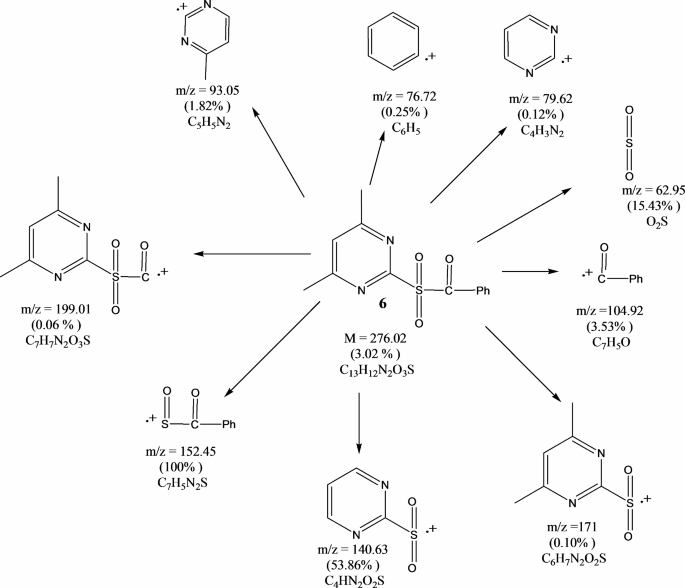
Mass fragmentations of compound 6.
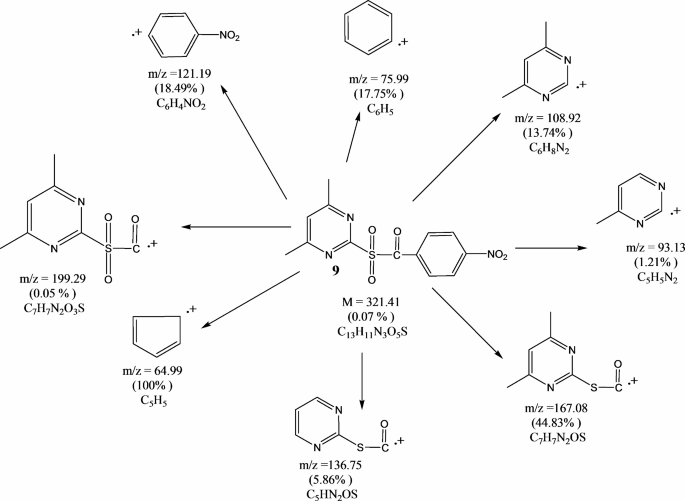
Mass fragmentations of compound 9.
Additionally, the reaction of compound 1 with sulfonyl chloride derivatives afforded pyrimidine sulfonothioate derivatives (10–12), which undergo sulfoxidation by hydrogen peroxide and glacial acetic acid to provide sulfonyl sulfonyl pyrimidine derivatives (13–15) (Fig. 5).
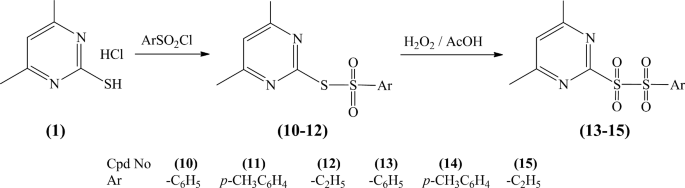
Sulfoxidation of Pyrimidine Sulfonothioate Derivatives.
Characterization of pyrimidine sulfonothioate derivatives (10–12)
Treatment of 4,6-dimethylpyrimidin-2-thiol hydrochloride (1) with benzene sulfonyl chloride, 4-methyl benzene sulfonyl chloride, and/or ethane sulfonyl chloride in pyridine gave (4,6-dimethylpyrimidin-2-yl)benzenesulfonothioate (10), (4,6-dimethylpyrimidin-2-yl)4-methyl benzenesulfono thioate (11) and/or (4,6-dimethylpyrimidin-2-yl)ethane sulfonothioate (12), respectively.
FT-IR spectra of compounds (10–12) showed sulfonyl groups at the range 1238–1325 cm−1 (Fig. 6). 1H-NMR spectrum (CDCl3) of compound 10 provided (s, 6H, pyrimidine methyl protons) at 2.22, (s, 1H, aromatic protons of pyrimidine ring) at 7.28 and (m, 5H, aromatic protons) at 7.35–8.82 ppm. 1H-NMR spectrum (CDCl3) of compound 11 illustrated (s, 3H, tolyl methyl protons) at 1.1, (s, 6H, pyrimidine methyl protons) at 2.44, (s, 1H, aromatic protons of pyrimidine ring) at 7.60 and (m, 4H, aromatic protons) at 7.31–7.88 ppm. 1H-NMR spectrum (CDCl3) of compound 12 showed the chemical shift (δ = ppm) at 1.00–1.18 (t, 3H, methyl protons of ethyl group), at 2.29 (s, 6H, pyrimidine methyl protons), at 4.29–4.35 (q, 2H, methylene protons of ethyl group) and 7.28 (s, 1H, aromatic protons of pyrimidine ring).
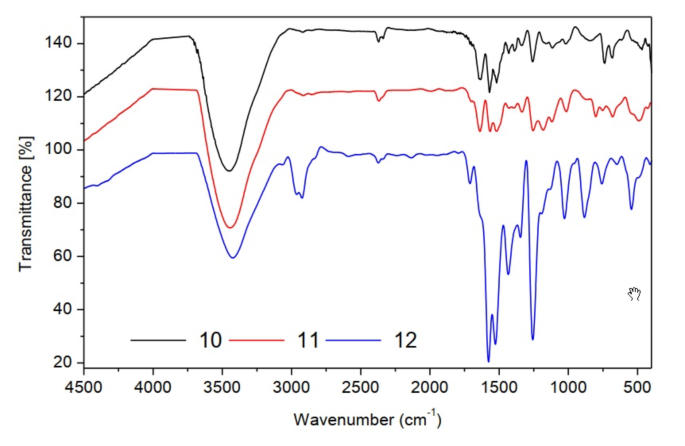
FT-IR spectra of pyrimidine sulfonothioate derivatives (10–12).
Characterization of sulfonyl sulfonyl pyrimidine derivatives (13–15)
The reaction of pyrimidine sulfonothioate derivatives (10–12) with hydrogen peroxide and glacial acetic acid provided 4,6-dimethyl-2-((phenylsulfonyl)sulfonyl)pyrimidine (13), 4,6-dimethyl-2-(tosylsulfonyl)pyrimidine (14) and/or 2-((ethylsulfonyl)sulfonyl)-4,6-dimethyl pyrimidine (15), respectively.
FT-IR spectra of compounds (13–15) showed sulfonyl groups in the range 1238–1325 cm−1 (Fig. 7). 1H-NMR spectrum (CDCl3) of compound 13 illustrated (s, 6H, pyrimidine methyl protons) at 2.50, (s, 1H, aromatic protons of pyrimidine ring) at 7.54 and (m, 5H, aromatic protons) at 7.47–7.92 ppm. 13C NMR (CDCl3) δ(ppm) of compound 13: 167.60, 160.46, 139.82, 132.82, 129.47, 127.39, 119.89 and 40.34. The mass spectrum of compound 13 (Fig. 8) showed the molecular ion peak at 312.44 (M+, 0.70%). 1H-NMR spectrum (CDCl3) of compound 14 provided (s, 3H, tolyl methyl protons) at 1.2, (s, 6H, pyrimidine methyl protons) at 2.47, (s, 1H, aromatic protons of pyrimidine ring) at 7.24 and (m, 4H, aromatic protons) at 7.00–8.16 ppm. 13C NMR (CDCl3) δ(ppm) of compound 14: 178.65, 171.51, 151.81, 146.82, 141.37, 138.57, 130.99, 34.34 and 30.34. The mass spectrum of compound 14 (Fig. 9) showed the molecular ion peak at 326.69 (M+, 0.51%). 1H-NMR spectrum (CDCl3) of compound 15 showed (t, 3H, methyl protons of ethyl group) at 1.10–1.28, (s, 6H, pyrimidine methyl protons) at 2.35, (q, 2H, methylene protons of ethyl group) at 3.89–4.05 and (s, 1H, aromatic protons of pyrimidine ring) at 7.32 ppm. 13C NMR (CDCl3) δ(ppm) of compound 15: 171.58, 164.57, 130.99, 45.33.82, 30.44 and 09.99.
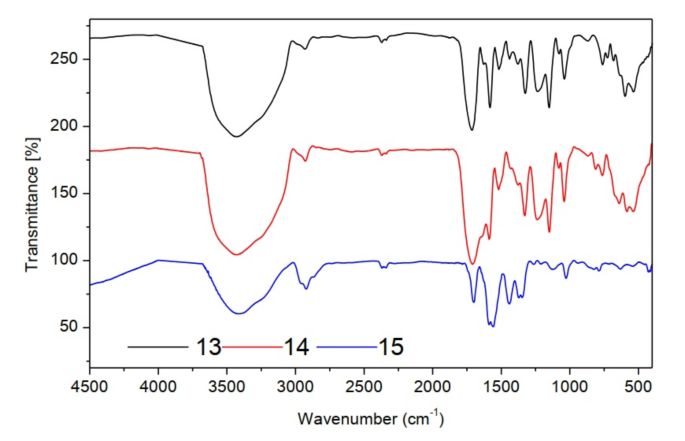
FT-IR spectra of sulfonyl sulfonyl pyrimidine derivatives (13–15).
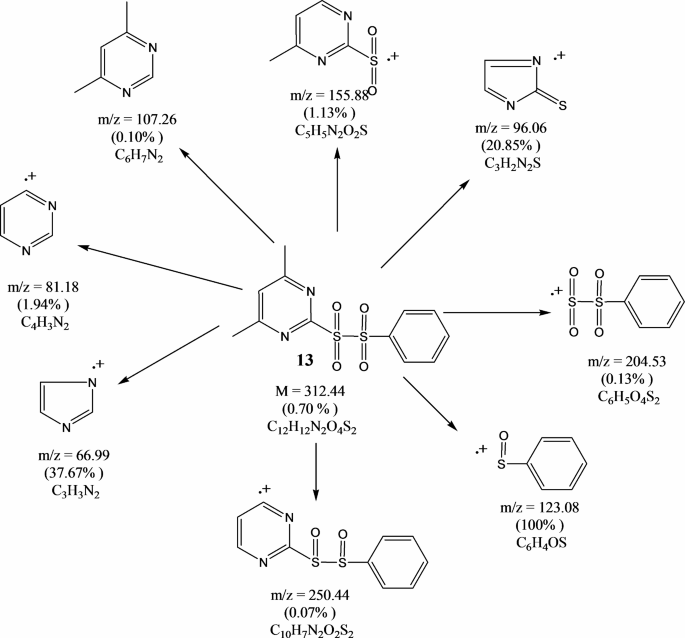
Mass fragmentations of compound 13.
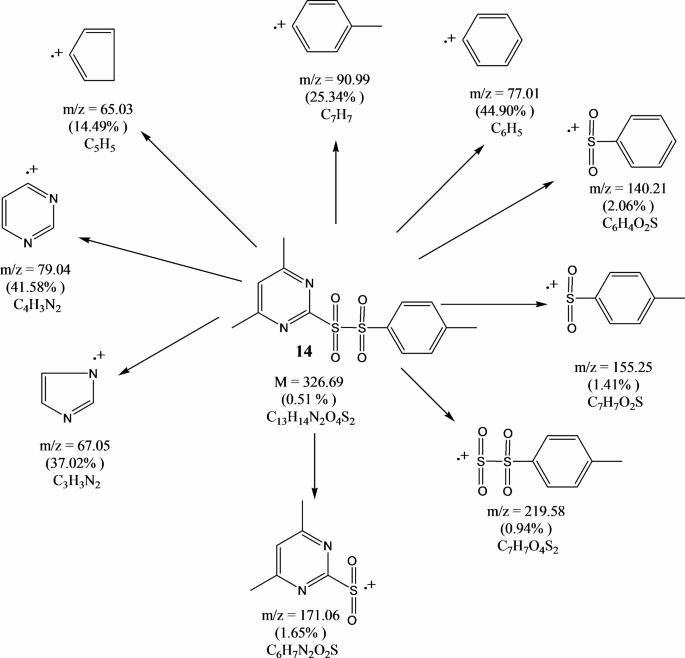
Mass fragmentations of compound 14.
The antibacterial activity of synthesized pyrimidine derivatives
The antimicrobial potential of the synthesized pyrimidine derivatives was evaluated against a selection of bacterial and fungal strains. The bacterial strains included human pathogenic strains Escherichia coli (EIEC), Escherichia coli (ATTC), Klebsiella pneumoniae, and Pseudomonas aeruginosa as Gram-negative bacteria. While the Gram-positive strains included Staphylococcus epidermidis, Staphylococcus haemolyticus, Methicillin-resistant Staphylococcus aureus (MRSA), and Proteus vulgaris. The fungal strain tested was Candida albicans. The study aimed to determine the antibacterial activity of the synthesized pyrimidine derivatives compared to the antibiotic floxacin and the antifungal activity compared to itraconazole, to assess their potential as antimicrobial agents (Table 2 and Fig. 10).
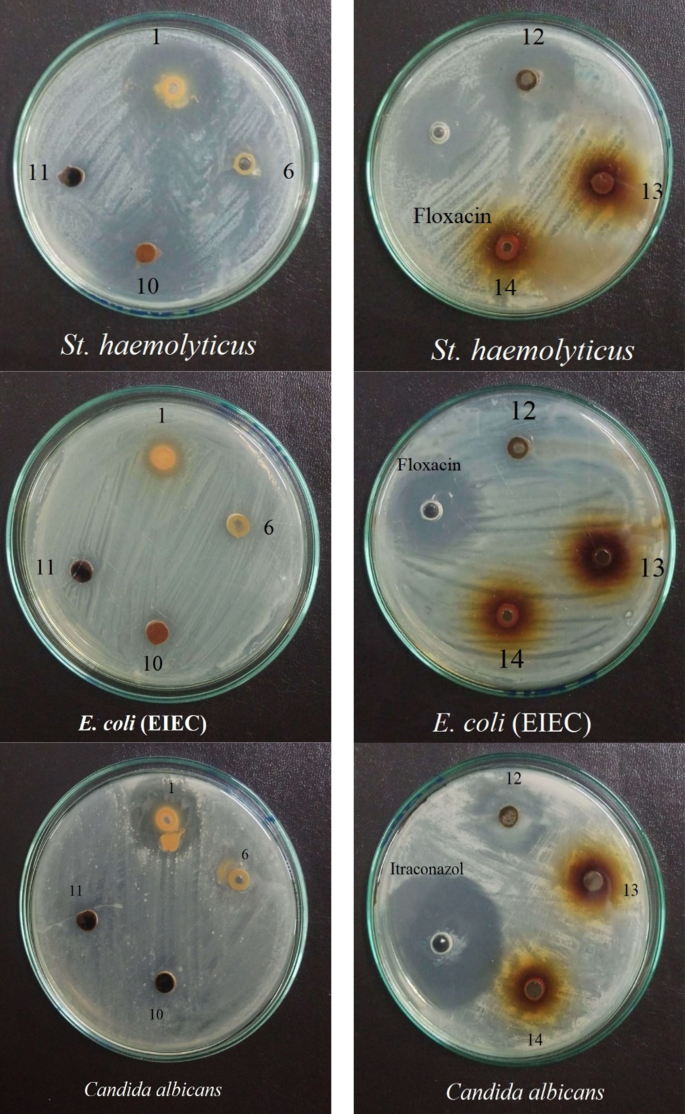
Representative images of the antimicrobial activity of the modified pyrimidine compounds.
Out of the synthesized pyrimidine derivatives, seven compounds 1, 6, 10, 11, 12, 13, and 14 were specifically examined for their antimicrobial effects. Compound 1 demonstrated significant antibacterial activity against all tested microorganisms, with inhibition zone diameters ranging from 13.67 to 31.67 mm. Notably, it displayed the highest activity against S. haemolyticus (31.67 mm), S. epidermidis (30.33 mm), and C. albicans (24.67 mm). However, it exhibited the least activity against E. coli EIEC (13.67 mm), P. vulgaris (14.33 mm), and E. coli ATTC (14.67 mm). Compound 6 showed antimicrobial activity against most of the tested microorganisms, except E. coli EIEC and P. vulgaris. Its most pronounced activity was observed against C. albicans, S. haemolyticus, and S. epidermidis, with inhibition zone diameters of 19.33, 17.00, and 14.33 mm, respectively.
Compound 10 did not exhibit antimicrobial activity against the tested Gram-negative bacteria or the fungal strain. However, it displayed potent antibacterial activity against S. haemolyticus, S. epidermidis, and MRSA, with inhibition zone diameters of 34.33, 20.33, and 18.33 mm, respectively. Compound 11 did not show any antimicrobial activity against any of the tested microorganisms. Similarly, compounds 12, 13, and 14 did not demonstrate antimicrobial efficacy against the tested Gram-negative bacteria or the unicellular yeast-fungal strain C. albicans, except for compound 12, which exhibited inhibition zones 25.33 mm against C. albicans. This compound also exhibited inhibition zones of 34.67, 30.67, and 18.67 mm against S. haemolyticus, S. epidermidis, and MRSA, respectively. Compound 13 only showed an antibacterial effect against S. epidermidis (20.33 mm), while compound 14 produced inhibition zone diameters of 22.00 and 17.33 mm against S. epidermidis and S. haemolyticus, respectively.
The antibiotic floxacin displayed relatively efficient antibacterial effects against all tested strains, with mean inhibition zone diameters ranging from 19.33 to 41.00 mm. Similarly, the antifungal agent itraconazole exhibited potent antifungal activity against C. albicans, with a mean inhibition zone of 45.00 mm. Additionally, the solvent used (DMSO) to prepare the compound solutions did not exhibit any antimicrobial effects against the tested microorganisms.
Pyrimidine derivatives have gained significant attention in the field of medicinal chemistry due to their diverse biological activities, including antimicrobial properties. Several studies have investigated the antimicrobial potential of pyrimidine derivatives against various microorganisms such as bacteria, fungi, and parasites29,30. Pyrimidine derivatives have shown promising antimicrobial effects against a wide range of bacteria, including both Gram-positive and Gram-negative strains. For instance, two studies evaluated the antimicrobial activity of some newly synthesized pyrimidine derivatives and found that they exhibited significant inhibitory effects against both methicillin-resistant Staphylococcus aureus (MRSA) and Escherichia coli strains31,32. Similarly, another study by Suresh et al.33 reported the synthesis of pyrimidine derivatives and demonstrated their potent antibacterial activity against Bacillus cereus and Staphylococcus aureus strains. Pyrimidine derivatives have also displayed notable antifungal activity against various fungal pathogens. In a study focusing on the antifungal activity of some newly synthesized pyrimidine derivatives, the evaluated compounds exhibited potent antifungal activity against Trichophyton rubrum, Aspergillus flavus, and Geotrichum candidum, indicating their potential as antifungal agents34. Furthermore, another study investigating the antifungal activity of pyrimidine derivatives has demonstrated their inhibitory effects against Aspergillus flavus, A. fumigatus, Candida albicans, and Penicillium marneffei strains35.
The antimicrobial activity of compound 1 was significantly reduced upon introducing modifications, namely the substitution by a benzoyl group (compound 6), phenyl group (compound 10), tolyl group (compound 11), or through sulfoxidation (compounds 13 and 14), as observed in the present investigation. The introduction of a benzoyl group (compound 6) likely disrupted the molecular arrangement necessary for effective interaction with microbial targets36. This substitution might have altered the physicochemical properties of the compound, affecting its ability to bind to specific receptors or enzymes crucial for microbial growth and survival. Similarly, the substitution with a phenyl group (compound 10) or tolyl group (compound 11) could have caused steric hindrance or changes in electronic properties, leading to a compromised interaction with target molecules involved in microbial processes37. These modifications may have hindered the compound’s ability to exert its antimicrobial effects effectively. Furthermore, the sulfoxidation process applied to compounds 13 and 14, which involves the introduction of an oxygen atom, may have altered the overall spatial arrangement and electronic characteristics of the molecules. This change could have disrupted the structural requirements for effective binding to microbial targets, resulting in reduced antimicrobial activity.
MIC of the most promising pyrimidine derivatives
The present study assessed the minimum inhibitory concentration (MIC) of the pyrimidine compounds to determine their effectiveness against the tested microorganisms. Various concentrations (7.5, 15, 30, and 60 mg/mL) of the compounds were prepared and tested against the pathogenic strains as outlined in (Table 3). For compound 1, S. epidermidis was inhibited at a concentration of 7.5 mg/mL, while K. pneumoniae, P. aeruginosa, S. haemolyticus and C. albicans were inhibited at a concentration of 15 mg/mL. In contrast, E. coli, MRSA, and P. vulgaris required a concentration of 30 mg/mL to achieve inhibition. Compound 6 exhibited lower efficacy compared to compound 1. It inhibited the growth of S. epidermidis, S. haemolyticus, and C. albicans at a concentration of 30 mg/mL, while the remaining organisms required a concentration of 60 mg/mL for inhibition.
At a concentration of 15 mg/mL, compound 10 inhibited the growth of S. haemolyticus, whereas S. epidermidis and MRSA required a concentration of 30 mg/mL for inhibition. Compound 12 inhibited S. haemolyticus at a concentration of 7.5 mg/mL, while a concentration of 15 mg/mL was sufficient to inhibit the growth of S. epidermidis and C. albicans. MRSA displayed higher resistance, requiring a concentration of 30 mg/mL for inhibition by compound 12. Similarly, compounds 13 and 14 exhibited the least efficiency in inhibiting S. epidermidis, as their growth was inhibited at a concentration of 30 mg/mL.
Radical scavenging (DPPH*) activity of the pyrimidine derivatives
The principle behind this test is to use electron withdrawal potential from the examined compound to bleach the violet color of the DPPH* radical. The more potential for donating electrons a compound has the greater radical scavenging activity it possesses. According to the findings of this study, compounds 12, and 6 showed higher scavenging activity than the start compound 1, as the modification made on these compounds, through ethyl and benzoyl substitution, had resulted in 127.56, and 51.10%, respectively, increases in the DPPH scavenging activity of these compounds as compared to the start compound (Table 4). Modification of the starting compound to produce compounds 13 and 14, on the other hand, resulted in a significant reduction in their radical scavenging activity, with compound 13 having the least activity. Contrastingly, the modification made on compounds 10 and 11 resulted in complete inhibition of the radical scavenging activity. One of the most used techniques for figuring out whether a natural or synthetic chemical molecule has antiradical and antioxidant characteristics is the DPPH radical scavenging test. The utilization of the pyrimidine derivatives as antioxidants and anti-inflammatory agents is just one of their many different applications.
Pyrimidine derivatives have demonstrated significant antioxidant activity, making them valuable compounds in the field of oxidative stress-related diseases. These derivatives possess the ability to scavenge reactive oxygen species (ROS) and inhibit oxidative damage to cellular components. The antioxidant activity of pyrimidine derivatives is primarily attributed to their structural features, such as the presence of electron-donating functional groups38. These groups facilitate the donation of electrons or hydrogen atoms to unstable ROS, thereby neutralizing their harmful effects. The derivatives’ antioxidant properties help maintain cellular redox balance and protect biomolecules, including lipids, proteins, and DNA, from oxidative damage. Furthermore, pyrimidine derivatives have shown the ability to chelate transition metal ions, such as iron and copper, which can contribute to the generation of harmful free radicals39. By chelating these ions, the derivatives prevent their participation in Fenton and Haber-Weiss reactions, which generate highly reactive hydroxyl radicals. These properties make them promising candidates for the development of therapeutic agents to combat oxidative stress-related diseases and conditions. Further research is needed to explore their full potential and optimize their efficacy as antioxidants.
The antitumor activity of some synthesized pyrimidine derivatives
One of the objectives of this study was to analyze the effectiveness of two newly synthesized pyrimidine derivatives, compounds 10 and 12, in inhibiting tumor growth in the MCF-7 breast carcinoma cell line. To evaluate their efficacy, the MTT assay was utilized, and the results were compared to those obtained with the standard antitumor drug cisplatin (Table 5 and Fig. 11). The findings indicated that compound 12 exhibited a minimal level of antitumor activity, as evidenced by its IC50 value of 420.75 µg/ml, suggesting relatively lower efficacy in inhibiting tumor growth. In contrast, compound 10 demonstrated moderate antitumor activity against the MCF-7 cell line, with an IC50 value of 195.40 µg/ml. Notably, when compared to cisplatin, which displayed an IC50 value of 5.69 µg/ml, compound 10 exhibited relatively moderate antitumor activity, thereby making it a promising candidate for further in vitro investigations to ascertain its potency in inhibiting tumor growth in other cell lines.
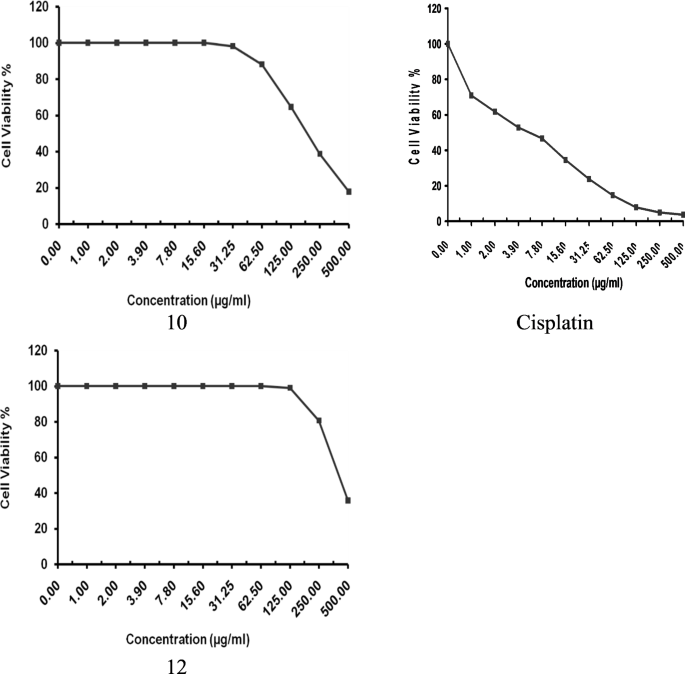
The antitumor activity of some synthesized pyrimidine compounds (10 and 12) against the breast cancer cell line MCF-7 as compared to cisplatin standard antitumor as detected using MTT assay.
Pyrimidine compounds have been reported as promising antitumor compounds, making them suitable anticancer candidates. Through various mechanisms, these compounds affect tumor development and progression processes. Pyrimidine derivatives block the DNA-synthesis enzyme thymidylate synthase (TS)19. These compounds impede TS, preventing DNA replication by blocking thymidine synthesis. This disruption damages DNA and inhibits cell division, preventing tumor growth. Pyrimidine derivatives also inhibit EGFR and VEGFR tyrosine kinases20,21. Tumor cell proliferation, angiogenesis, and metastasis depend on these kinases. Pyrimidine derivatives block these signaling pathways, preventing cancer cell proliferation and blood vessel development22. Additionally, several pyrimidine derivatives were reported to induce cancer cell death by activating programmed cell death, as they trigger apoptotic pathways that eradicate cancer cells23. These properties highlight their potential as targeted therapies against various types of cancers, although further research and clinical trials are necessary to fully explore their efficacy and optimize their therapeutic potential.
Structure activity relationship (SAR) of the synthesized compounds
The outcomes of biological assays conducted on the synthesized derivatives of 4,6-dimethylpyrimidine-2-thiol hydrochloride offer valuable insights into the Structure–Activity Relationship (SAR) of these compounds. Specifically, Compound 1, featuring a thiol group on the pyrimidine ring, exerts a substantial influence on the compound’s antimicrobial efficacy. Thiol groups are recognized for their adeptness in engaging with a spectrum of biological entities, encompassing proteins and enzymes, thereby impacting microbial proliferation and viability40. Moreover, the hydrophobic characteristics of the thiol group play a pivotal role in modulating the compound’s capacity to infiltrate microbial membranes, thereby facilitating its ingress into microbial cells. These thiol groups can establish covalent bonds with pivotal enzymes crucial for microbial sustenance, thereby impeding their functionality and disrupting the microbial redox equilibrium, consequently bolstering the antimicrobial properties of the compound. The SAR observations concerning compounds 6 and 10 underscore how the phenyl ring can augment the antifungal and antibacterial efficacy of the synthesized pyrimidine derivatives4. Similarly, the SAR analysis of compound 12 delineates how the introduction of an ethyl group can enhance the antifungal and antibacterial effectiveness of the synthesized pyrimidine derivatives. Conversely, the restricted antimicrobial performance of compound 14 elucidates that the inclusion of p-tolyl may compromise the efficacy of the pyrimidine derivatives against the targeted pathogens.
Furthermore, owing to the radical scavenging attributes of the synthesized compounds, it is evident that compound 12 (ethyl substitution) and compound 6 (phenyl substitution) exhibit heightened scavenging activity compared to the start compound. The incorporation of an ethyl group into the pyrimidine ring is noted to enhance radical scavenging proficiency due to the electron-donating capabilities of ethyl groups, which potentially enhance the compound’s ability to counteract radicals. Similarly, the addition of a phenyl group elevates radical scavenging efficacy owing to the aromatic nature of the phenyl group, which bolsters the compound’s stability and reactivity towards radicals. Additionally, phenyl groups can facilitate electron delocalization, thereby influencing the radical scavenging capabilities of the compound.
Regarding anti-tumor effectiveness, compound 10 has demonstrated notable potency against the MCF-7 cell line, demonstrating moderate anti-tumor activity relative to cisplatin. The SAR analysis of pyrimidine sulfonothioate phenyl underscores the significance of substituting a phenyl group onto a pyrimidine scaffold, coupled with a sulfonothioate moiety. While the sulfonothioate group endows reactivity and specificity, the phenyl group contributes to lipophilicity and target affinity41,42. By comprehending the SAR intricacies of the modified pyrimidine derivatives, researchers can optimize their designs to elicit desired biological effects, spanning antimicrobial, antioxidant, and anticancer activities, through precise structural modifications and property tunings.
Leave a Reply